The Impact of Quantum Physics on Computing
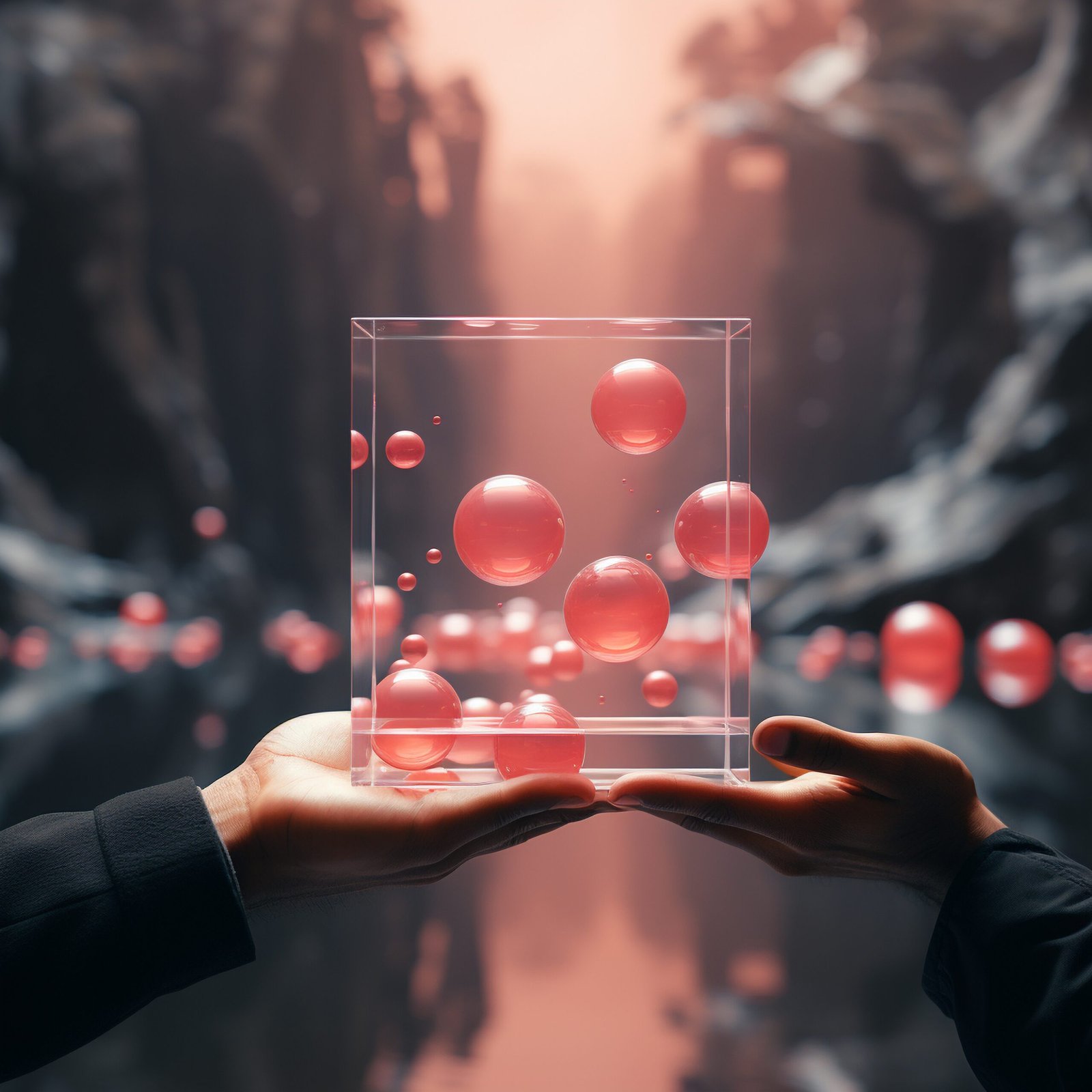

by Web Digital
Quantum physics, the mind-bending science that explores the behaviour of matter and energy at the smallest scales, has transcended the realm of theoretical physics and made its mark on the world of computing. In recent years, quantum computing has emerged as a groundbreaking field with the potential to revolutionize the way we process information. At the heart of this transformation are quantum bits (qubits) and quantum gates. This article explores the impact of quantum physics on computing and the fascinating world of qubits and quantum gates.
Classical vs. Quantum Computing
To understand the significance of quantum bits and quantum gates, it’s essential to distinguish between classical and quantum computing.
1. Classical Computing
Classical computers, the devices we use daily, process data using bits as the fundamental unit of information. Bits can represent either a “0” or a “1. Classical computers perform operations by manipulating sequences of 0s and 1s through logical gates (AND, OR, NOT, etc.). While classical computers are incredibly powerful, they face limitations when it comes to solving certain complex problems efficiently.
2. Quantum Computing
Quantum computing takes a radically different approach. Instead of using classical bits, it employs quantum bits or qubits. Unlike classical bits, qubits can exist in multiple states simultaneously, thanks to the principle of superposition. This means that a qubit can represent either a “0,” a “1,” or any combination of “0” and “1” simultaneously. Additionally, qubits can be entangled, a phenomenon where the state of one qubit is dependent on the state of another, even if they are separated by great distances.
Quantum Bits (Qubits)
The foundational elements of quantum computing are qubits. They possess unique properties that set them apart from classical bits and enable quantum computers to tackle complex problems more efficiently.
1. Superposition
Superposition allows qubits to represent multiple states simultaneously. For example, a classical bit can be either 0 or 1, while a qubit can be 0, 1, or any combination of both. This property enables quantum computers to perform multiple calculations in parallel, significantly speeding up certain tasks.
2. Entanglement
Entanglement is a phenomenon where the state of one qubit is intrinsically linked to the state of another, even when they are physically separated. This property enables quantum computers to perform operations on entangled qubits, providing a powerful means of solving complex problems, such as those in cryptography and optimization.
3. Quantum Interference
Quantum interference is another crucial property of qubits. It allows quantum computers to manipulate the probabilities of different outcomes, increasing the likelihood of obtaining the correct answer in certain computations.
Quantum Gates
In classical computing, logical gates (e.g., AND, OR, XOR) perform operations on bits to carry out tasks like addition, subtraction, and logical comparisons. In quantum computing, the equivalent of these gates is quantum gates.
1. Quantum NOT Gate (X Gate)
The quantum NOT gate, often represented as the X gate, flips the state of a qubit. If the qubit is in the state |0⟩, it becomes |1⟩, and vice versa.
2. Quantum Hadamard Gate (H Gate)
The Hadamard gate creates superposition by transforming a |0⟩ qubit into a state that is an equal superposition of |0⟩ and |1⟩. It is a fundamental gate for creating quantum entanglement.
3. Quantum CNOT Gate
The controlled NOT gate, or CNOT gate, is a two-qubit gate that performs an operation on one qubit (the target) based on the state of another qubit (the control). It plays a crucial role in creating entanglement and implementing quantum algorithms.
4. Quantum Phase Gate
The quantum phase gate is used to manipulate the phase of a qubit’s state. It is essential for quantum algorithms like the Quantum Fourier Transform, a key component of Shor’s algorithm for factoring large numbers—a task that poses a significant challenge for classical computers.
Quantum Computing Applications
Quantum computing’s potential extends to various fields, including:
1. Cryptography
Quantum computers have the potential to break widely used encryption algorithms like RSA and ECC. Consequently, they also hold the promise of creating unbreakable quantum-safe encryption methods.
2. Drug Discovery
Quantum computers can simulate molecular interactions with unprecedented accuracy, greatly expediting the drug discovery process and potentially leading to the development of new treatments for diseases.
3. Optimization
Problems like route optimization, resource allocation, and supply chain management can be solved much more efficiently with quantum algorithms, potentially saving significant time and resources.
4. Artificial Intelligence
Quantum computing has the potential to enhance machine learning algorithms, allowing for more rapid analysis of complex datasets and solving problems that are currently beyond the capabilities of classical computers.
Challenges and Considerations
While quantum computing offers tremendous promise, it also presents challenges:
1. Error Correction
Quantum bits are highly susceptible to errors due to factors like decoherence and environmental noise. Developing robust error correction techniques is a critical area of research.
2. Scalability
Building large-scale quantum computers with thousands or millions of qubits is a formidable engineering challenge. Current quantum computers are relatively small, and achieving scalability is essential for realizing their full potential.
3. Algorithm Development
Designing quantum algorithms that harness the power of quantum computing for practical applications is a complex task. The foundational elements of quantum computing are qubits.
4. Quantum Advantage
Determining the specific problems for which quantum computers offer a significant advantage over classical computers remains an ongoing research question.
Conclusion
While quantum computing is still in its infancy and faces numerous challenges, its potential to transform fields ranging from cryptography to drug discovery is undeniable. As researchers and engineers continue to push the boundaries of quantum technology, we are on the cusp of a computing revolution that promises to reshape our understanding of computation and revolutionize the way we tackle some of the world’s most pressing challenges. The impact of quantum physics on computing is not just a theoretical concept; it’s a glimpse into the future of technology and innovation.
Recommended Posts

Non-Profit Digital Marketing in Wasaga Beach: Maximizing Impact
December 5, 2024